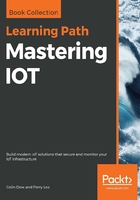
RF energy and theoretical range
It is important when talking about wireless personal area networks, or any RF wireless protocol, to consider the range of transmission. Competing protocols use range, speed, and power as differentiators. As architects, we need to consider different protocols and design choices when implementing a complete solution. The range of transmission is based on the distance between transmitter and receiver antennas, the frequency of transmission, and the power of transmission.
The best form of RF transmission is unobstructed line-of-sight in a radio signal-free area. In most situations, this ideal model will not exist. In the real world, there are obstructions, reflections of signals, multiple wireless RF signals, and noise.
When considering a particular WAN and either a slower speed signal like 900 MHz versus a 2.4 GHz carrier signal, you can derive the attenuation of a function of wavelength for each frequency. This will provide guidance as to the signal strength at any range. The general form of the Friis transmission equation helps us here:

The decibel (dB) variant of the Friis equation is given as:

Where GTx and GRx are the transmitter and receiver antenna gains, R is the distance between the transmitter and receiver, and PR and PT are the receiver and transmitter power respectively.

A 900 MHz signal at 10 meters will have a loss of 51.5 dB and a 2.4 GHz signal at 10 meters will have a 60.0 dB loss.
We can prove how power and range affect the quality of a signal by using a ratio referred to as the link budget. This is a comparison of the transmission power to sensitivity level and is measured in the logarithmic (dB) scale. One may simply want to increase power level to meet range requirements, but in many instances, this violates regulatory compliance or affects battery life. The other option is to improve the receiver's sensitivity level, which is exactly what Bluetooth 5 did in the latest specification. The link budget is given by the ratio of transmitter power over the receiver sensitivity as shown.

The link budget is measured in the dB log scale; therefore, adding decibels is equivalent to multiplying the numeric ratios, which give us the equation:

Assuming there is no factor contributing to any signal gains (for example, antenna gains), the only two ways of improving reception is increasing the transmission power or decreasing the loss.
When an architect must model the maximum range of a particular protocol, he or she will use the Free Space Path Loss (FSPL) formula. This is the amount of signal loss of an electromagnetic wave over a line of sight in free space (no obstacles). The contributing factors of FSPLF are the frequency (f) of the signal, the distance (R) between transmitter and receiver, and the speed of light (c). In terms of calculating FSPLF in decibels, the equation is:

The FSPL formula is a simple first-order calculation. A better approximation takes into account reflections and wave interference from the earth ground plane, such as the plane earth loss formula. Here, ht is the height of the transmitting antenna and hr is the height of the receiving antenna. k represents the free space wave number, and is simplified as shown. We convert the equation to use dB notation:

What is notable of the plane earth loss is the fact that the distance affects the loss by 40 dB per decade. Increasing the antenna height helps. The types of interference that can naturally occur include:
- Reflections: When a propagating electromagnetic wave strikes an object and results in multiple waves
- Diffraction: When a radio wave path between a transmitter and receiver is obstructed by objects with sharp edges
- Scattering: When the medium the wave travels through is comprised of objects smaller than the wavelength and the number of obstacles is large
This is an important concept since the architect must choose a WAN solution whose frequency balances the data bandwidth, the ultimate range of the signal, and the ability of the signal to penetrate objects. Increasing frequency naturally increases free space loss (for example, a 2.4 GHz signal has 8.5 dB less coverage than a 900 MHz signal). Generally speaking, 900 MHz signals will be reliable at twice the distance of 2.4 GHz signals. 900 MHz has a wavelength of 333 mm versus 125 mm for a 2.4 GHz signal. This allows a 900 MHz signal to have better penetration ability and not as much effect from scattering.
Scattering is a significant issue for WAN systems as many deployments do not have a free line of sight between antennas—instead the signal must penetrate walls and floors. Certain materials contribute differently to the attenuation of a signal.
We see that 900 MHz has an advantage over 2.4 GHz in material penetration:


As we will see, many protocols are commercially available and used globally in the 2.4 GHz spectrum. 2.4 GHz provides up to five times the data bandwidth as a 900 MHz signal and can have a much smaller antenna. Additionally, the 2.4 GHz spectrum is unlicensed and available for use in multiple countries.

These equations provide a theoretical model; no analytical equations give an accurate prediction for certain real-world scenarios such as multi-path losses.