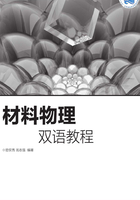
2.1 Bonding Modes Among Atoms
The structural elements of matter are the atoms, which consist of a nucleus and the atomic shell. The properties of solids are essentially determined by the electron shell structure. According to the Bohr model of an atom, the electrons occupy specific orbitals (Figure 2.1), the configuration of which, i.e. number of electrons and their spatial arrangement, follows the laws of quantum mechanics. The most important electrons for the properties of a solid are the electrons in the outermost orbital, because they determine the interaction with other atoms. The dominant principle of atomic interaction is the tendency of an atom to have its outermost shell filled with eight electrons, i.e. the noble gas configuration. This simple principle is the foundation of chemical bonding. If an atom has already a complete outer shell with eight electrons, like the noble gases, then its tendency to interact with other atoms, i.e. for chemical bonding or even for solidification is very small. All elements which do not have a noble gas configuration have the tendency to accept, to donate, or to share the outermost electrons, also referred to as valence electrons, when in contact with other atoms. From these principles we obtain the fundamental types of atomic bonding. An understanding of this helps categorize materials as metals, nonmetals, organics, or inorganics, etc. It also permits us to draw some general conclusions concerning material properties. There are four types of atomic bonding in materials: metallic bonding, ionic bonding, covalent bonding, and molecular bonding.

Figure. 2.1 Diagrammatic illustration of the electronic configuration of the 12C atom after Bohr's atomic model.
• Metallic bond
As shown in Figure 2.2b, If the number of valence electrons is less than four, a noble gas configuration cannot be established by electron pairs in a three dimensional lattice. In this case the atoms prefer to donate their valence electrons to a common electron gas and form the metallic bond. So that the ionic cores attain the noble gas configuration and the electrons in the electron gas are not associated with a particular atom. The metallic bond is essentially coulombic, but the negatively charged species are the electrons that are delocalized and free to move throughout the solid. This is the most frequent type of bond among the elements, because about three quarters of all natural elements are metals. In the metallic bond the electrons are not localized and practically belong to all atoms at the same time. The weak localization of the electrons in the metallic bond is the reason for its weakness in comparison to the other types of bonds. In turn, this causes the high mobility of defects in metals and, therefore, their excellent formability, which has made metals the dominant structural materials. The electrical conductivity and reflectivity are quite high, and the melting point is high, although it tends to be lower than that of the ionic solids. Metals exhibit a characteristic malleability and ductility.

Figure 2.2 (a) Stacking of metallic atoms. (b) Metallic bonding.
• Ionic bond
An ionic bond is built between two unlike atoms with different electronegativity. The number of valence electrons of the partners adds up to eight. The partner with smaller valency donates its valence electrons to the other partner with higher valence. Both elements attain a noble gas configuration, but the atoms lose their charge neutrality. For instance, in sodium chloride when sodium donates its valence electron to chlorine, each atom becomes an ion, coulombic attraction occurs, forming the ionic bond (Figure 2.3). Ionic solids tend to have relatively high melting points(sodium chloride melts at 801 ℃) and are rather brittle. They are not good electronic conductors at low temperatures and exhibit ionic conductivity at temperature close to and above the melting point.

Figure 2.3 (a) Atom stacking in NaCl. (b) Ionic bond of NaCl.
• Covalent bond
If the noble gas configuration cannot be established by exchange of electrons since the sum of valence electrons does not add to eight, a stable arrangement of atoms in a molecule can be obtained by the formation of electron pairs. A covalent solid is formed and characterized by bonding that results from the sharing of electrons between neighboring atoms (Figure 2.4); the bonding can have a polar character. Diamond, silicon carbide, quartz, silicon, and germanium are examples of covalently bonded solids. These solids tend to have a low electronic conductivity and high melting point and are likely to be hard and brittle.

Figure 2.4 (a) Covalent bonding requires that electrons be shared between atoms in such a waythat each atom has its outer sp orbitals filled. (b) In silicon, with a valence of four, four covalentbonds must be formed. (c) Covalent bonds are directional. In silicon, a tetrahedral structure isformed with angles of 109.5° required between each covalent bond.
• Molecular bond
The previously discussed three bondings are primary bonding, i.e., the atomic (chemical) bonding. In materials of some cases, there is a secondary bonding, namely the so-called Van der Waals bond, which is not associated with the donating-accepting or share of electrons but caused by a dipolar(either an induced or permanent dipolar) interaction of atoms. This dipole interaction is caused by the fact that the center of gravity of charge of the nucleus is not identical with that of the electron shell. This causes a dipole moment of the atoms which invariably generates an attractive interaction with other atomic dipoles. This attractive force is the reason for bonding in noble gas molecules and the interaction of far apart atoms, when no exchange of electrons can take place.
Molecular solids are composed of discrete molecules held together by weak van der Waals forces; though the molecules themselves can consist of atoms held together by covalent bonds. Van der Waals forces between atoms and molecules have their origin in interactions between dipoles that are induced or in some cases interactions between permanent dipoles that are present in certain polar molecules. There are three types of van der Waals interactions, namely London force, Keesom force and Debye force.
If the interactions are between two dipoles that are induced in atoms or molecules (e.g., carbon tetrachloride), we refer to them as London forces, which are also termed as dispersive force. Figure 2.5 illustrates this type of force.

Figure 2.5 Illustration of London forces, a type of a van der Waals force, between atoms.
When an induced dipole (that is, a dipole that is induced in what is otherwise a non-polar atom or molecule) interacts with a molecule that has a permanent dipole moment, we refer to this interaction as a Debye interaction (Figure 2.6).

Figure 2.6 Debye interaction between HCl and Ar molecules.
If the interactions are between molecules that are permanently polarized, it is referred as Keesom interactions. For instance, the positively charged region of one water molecule and the negatively charged regions of a second water molecule render an attractive bond between them(Figure 2.7).

Figure 2.7 Dipole-dipole interaction (Keesom force) between water molecules.
The bonding between molecules that have a permanent dipole moment is often referred to as a hydrogen bond, where hydrogen atoms represent one of the polarized regions. Thus, hydrogen bonding is essentially a Keesom force and is a type of van der Waals force.
Note that Van der Waals bonds are secondary bonds, but the atoms within the molecule or group of atoms are joined by strong covalent or ionic bonds. Although termed "secondary," based on the bond energies, van der Waals forces play a very important role in many material properties. First of all, because of the weak nature of the bonding between molecules in a molecular solid, the energy orbitals between molecules cannot form band structure, but exist isolated, leading to low conductivity. So the molecular solids exhibit narrow band width, wide bandgap and low carrier mobility. It is to be expected that the properties of the individual molecules are retained in the solid state to a far greater extent than in solids with other three types of bonding. Secondly, due to the weak interaction among molecules, molecular solids are soft with low melting points. In addition, low interaction renders loose stack of molecules and large lattice variation. Especially, factors such as temperature, pressure, and external magnetic field can change the space arrangement of molecular solid easily, which results in change of material properties. Specifically, van der Waals forces between atoms and molecules play a vital role in determining the surface tension and boiling points of liquids.
In many plastic materials, molecules contain polar parts or side groups (e.g., cotton or cellulose, PVC, Teflon). Van der Waals forces provide an extra binding force between the chains of these polymers. Polymers in which van der Waals forces are stronger tend to be relatively stiffer and exhibit relatively higher glass transition temperatures (Tg). The glass transition temperature is a temperature below which some polymers tend to behave as brittle materials (i.e., they show poor ductility). As a result, polymers with van der Waals bonding (in addition to the covalent bonds in the chains and side groups) are relatively brittle at room temperature (e.g. PVC). In handling such polymers, they need to be "plasticized" by adding other smaller polar molecules that interact with the polar parts of the long polymer chains, thereby lowering the Tg and enhancing flexibility.